Introduction
Quantum computing, a revolutionary paradigm in the world of computation, holds immense promise for tackling complex problems that have stymied classical computers for decades. Unlike classical computers that rely on bits as the fundamental unit of information (0 or 1), quantum computers use quantum bits or qubits, which can exist in multiple states simultaneously, thanks to the principles of superposition and entanglement. This article explores the significance of quantum computing in solving complex problems, the underlying principles of quantum mechanics, recent advancements in the field, and a wide range of problem domains where quantum computing has the potential to make a transformative impact.
-
Quantum Mechanics: The Foundation of Quantum Computing
1.1 Understanding Quantum Bits (Qubits)
At the heart of quantum computing are qubits, the quantum equivalent of classical bits. However, qubits possess unique properties that enable them to store and process information differently:
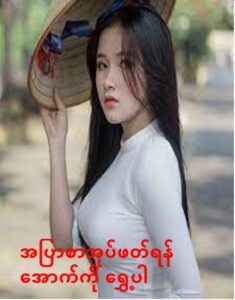
- Superposition: Unlike classical bits, which can only be in a state of 0 or 1, qubits can exist in a superposition of both states simultaneously. This property allows quantum computers to consider multiple possibilities in a single computation.
- Entanglement: Qubits can be entangled, meaning the state of one qubit is correlated with the state of another, even when they are separated by great distances. This property enables quantum computers to perform highly correlated operations efficiently.
1.2 Principles of Quantum Computing
Quantum computing leverages the principles of quantum mechanics to perform calculations:
- Quantum Gates: Quantum computers use quantum gates to manipulate qubits. These gates, analogous to classical logic gates, perform operations that exploit superposition and entanglement.
- Quantum Algorithms: Quantum algorithms are designed to harness the power of qubits to solve specific problems more efficiently than classical algorithms. Examples include Shor’s algorithm for factoring large numbers and Grover’s algorithm for searching unsorted databases.
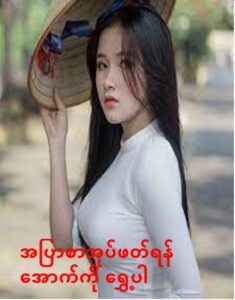
-
Quantum Computing Advancements
2.1 Quantum Hardware Development
Recent years have witnessed substantial progress in the development of quantum hardware:
- Quantum Volume: A metric used to quantify quantum computing capabilities, quantum volume has seen significant growth. Quantum computers with higher quantum volumes can handle more complex problems.
- Superconducting Qubits: Superconducting qubits, which are based on Josephson junctions, are among the leading technologies for building quantum processors. Improvements in fabrication techniques have led to more stable and coherent qubits.
- Ion Trap and Photonic Quantum Computers: Other technologies, such as ion trap and photonic-based quantum computers, have also made significant strides. Ion trap devices have achieved longer coherence times, while photonic quantum computers have demonstrated impressive qubit counts.
2.2 Quantum Error Correction
Quantum error correction codes are essential for addressing the inherent noise and errors in quantum hardware. Recent research has led to the development of more efficient quantum error correction codes, such as surface codes and cat codes. These codes help protect quantum information and improve the overall reliability of quantum computations.
2.3 Quantum Algorithms and Software
Advancements in quantum algorithms and software are enabling researchers to tackle increasingly complex problems:
- Quantum Machine Learning: Quantum machine learning algorithms leverage quantum properties to accelerate tasks like data classification, optimization, and matrix inversion. Variational quantum algorithms, such as the Quantum Approximate Optimization Algorithm (QAOA), show promise in various machine learning applications.
- Quantum Simulation: Quantum computers excel at simulating quantum systems, a task that is notoriously challenging for classical computers. Quantum simulations have applications in materials science, drug discovery, and understanding fundamental physics.
- Shor’s Algorithm and Cryptography: Although a threat to classical cryptography, Shor’s algorithm for integer factorization remains a focus of research. Quantum-resistant cryptographic algorithms, such as lattice-based cryptography, are being developed to counter the threat.
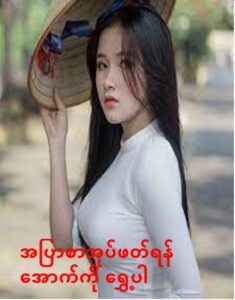
III. Problem Domains Addressed by Quantum Computing
Quantum computing’s significance lies in its potential to solve complex problems across various domains that are currently beyond the reach of classical computers:
3.1 Cryptography and Cybersecurity
- Breaking Current Cryptosystems: Quantum computers have the potential to break widely used public-key encryption schemes like RSA and ECC by efficiently factoring large numbers. This poses a significant threat to cybersecurity.
- Quantum-Safe Cryptography: Researchers are developing post-quantum cryptographic algorithms that are secure against quantum attacks. Quantum-safe cryptography will be crucial for safeguarding data and communications in a quantum-powered world.
3.2 Drug Discovery and Material Science
- Quantum Chemistry Simulations: Quantum computers can accurately simulate molecular structures and quantum interactions, allowing for accelerated drug discovery and the design of new materials with unique properties.
- Drug Optimization: Quantum algorithms can optimize the molecular structure of drugs, leading to more effective and personalized medications. This could revolutionize healthcare and drug development.
3.3 Optimization Problems
- Combinatorial Optimization: Quantum computing can provide efficient solutions to combinatorial optimization problems, such as the Traveling Salesman Problem (TSP), which has applications in logistics, supply chain management, and route optimization.
- Portfolio Optimization: Quantum computers can optimize investment portfolios, balancing risk and return by analyzing a vast number of asset combinations in real-time.
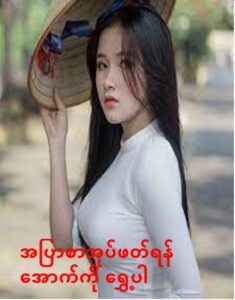
3.4 Artificial Intelligence and Machine Learning
- Quantum Machine Learning: Quantum algorithms have the potential to speed up machine learning tasks, including pattern recognition, recommendation systems, and optimization problems.
- Quantum Neural Networks: Quantum neural networks, a quantum analogue of classical neural networks, could unlock new capabilities in deep learning and artificial intelligence.
3.5 Climate Modeling and Energy Optimization
- Climate Simulation: Quantum computers can simulate complex climate models more accurately, aiding in climate prediction, carbon capture, and environmental policy planning.
- Energy Grid Optimization: Quantum optimization algorithms can optimize energy grids, helping to balance supply and demand and reduce energy waste.
3.6 Financial Modeling and Risk Analysis
- Portfolio Risk Analysis: Quantum computing can provide real-time risk analysis for financial portfolios, improving risk management in investment and trading.
- Option Pricing: Quantum algorithms can calculate option prices and risk exposures more efficiently, benefiting the financial industry.
3.7 Supply Chain Management
- Supply Chain Optimization: Quantum computing can optimize supply chain logistics, reducing costs and minimizing delays by solving complex routing and scheduling problems.
3.8 Quantum Sensing and Imaging
- Quantum Radar: Quantum radar systems could offer unprecedented capabilities in target detection and stealth technology.
- Quantum Imaging: Quantum sensors can capture images with enhanced precision and sensitivity, benefiting medical imaging, remote sensing, and security applications.
-
Challenges and Considerations
Despite the promising advancements and potential problem-solving capabilities, quantum computing faces several challenges and considerations:
4.1 Error Correction
Quantum hardware remains susceptible to errors and noise. Developing effective quantum error correction techniques is essential for building reliable quantum computers.
4.2 Scalability
Scaling quantum computers to handle larger problem sizes and qubit counts is a significant technical challenge. Maintaining qubit coherence and reducing error rates at scale is crucial.
4.3 Quantum-Resistant Cryptography
As quantum computing advances, the need for quantum-resistant cryptographic standards becomes increasingly urgent to protect sensitive data from quantum attacks.
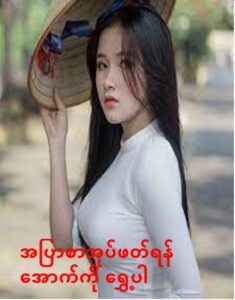
4.4 Resource Requirements
Quantum computing typically requires specialized infrastructure, including ultra-low-temperature environments and complex control systems. These requirements can be costly and limit accessibility.
4.5 Competition and Collaboration
The field of quantum computing is highly competitive, with various companies and research institutions vying for leadership. However, collaboration is essential for addressing common challenges and accelerating progress.
-
Quantum Computing’s Societal Impact
The widespread adoption of quantum computing will have a profound impact on society, affecting various aspects of our lives:
5.1 Security and Privacy
Quantum computers capable of breaking current encryption standards could compromise the security and privacy of digital communications and sensitive data. The transition to quantum-resistant cryptography will be critical to mitigate these risks.
5.2 Healthcare and Drug Discovery
Quantum computing can accelerate drug discovery and enable personalized medicine, potentially revolutionizing healthcare by improving treatment efficacy and reducing costs.
5.3 Climate Change Mitigation
Quantum simulations can enhance climate modeling and aid in the development of solutions for mitigating climate change, such as efficient carbon capture technologies.
5.4 Economic Implications
Quantum computing could reshape industries, create new markets, and impact employment. Businesses that harness quantum computing may gain a competitive advantage.
5.5 Ethical Considerations
As quantum computing capabilities advance, ethical considerations around data privacy, the responsible use of technology, and the potential misuse of quantum computing power will become increasingly important.
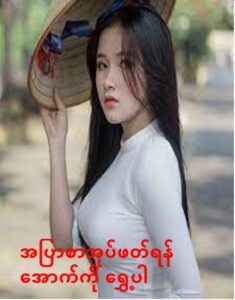
-
Conclusion
Recent advancements in quantum computing have propelled the field closer to realizing its transformative potential. Quantum computers, with their ability to perform complex calculations at speeds unattainable by classical computers, hold the promise of revolutionizing cryptography, drug discovery, optimization, materials science, and various other domains.
However, quantum computing is not without its challenges, including error correction, scalability, and the need for quantum-resistant cryptography. The societal impact of quantum computing will be profound, reshaping industries, security practices, and our approach to solving some of the world’s most pressing problems.
As quantum computing continues to progress, collaboration among researchers, industry stakeholders, and policymakers will be essential to navigate the opportunities and challenges that lie ahead. Quantum computing is not just a technological advancement; it represents a quantum leap in our understanding of computation and its potential to transform our world.